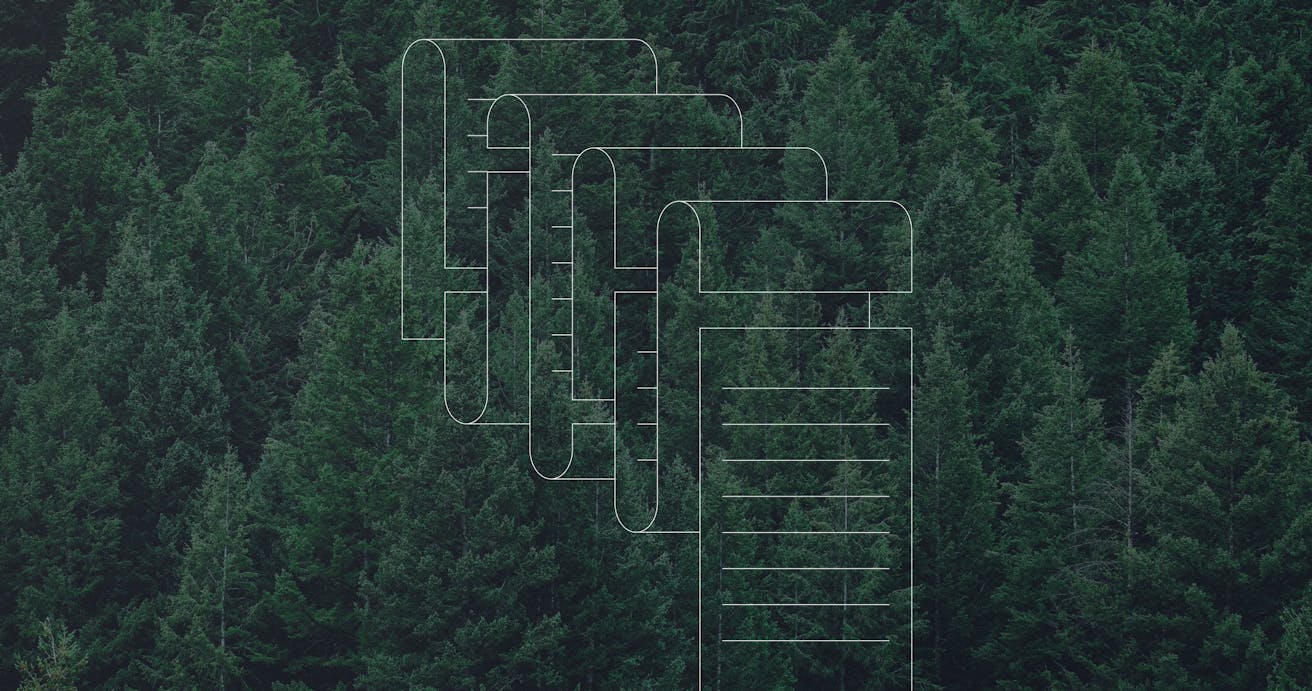
Environmental impact of digitalisation in shipping documentation: Carbon footprint comparison of paper and electronic bills of lading
Authors: Borut Istinič, Tadeja Škrjanec
ABSTRACT
The carbon footprint, an important measure of our contribution to climate change, helps us to understand and mitigate the impact of our activities. By measuring and managing our carbon footprint, we can promote a more sustainable and environmentally friendly future. In this paper, we aim to show that the electronic bill of lading (eBL) has a lower carbon footprint than its paper counterpart and therefore contributes to a reduction in environmental impact. Our study shows that electronic bills of lading can reduce carbon emissions by up to 96% compared to traditional paper bills of lading, depending on the blockchain technology used. Our results show that the transition from paper-based to electronic bills of lading leads to a significant reduction in the carbon footprint associated with the lifecycle of each document.
1. Introduction
The global shipping industry, responsible for approximately 90% of world trade [1], faces mounting pressure to reduce its environmental footprint [2]. While much attention has been given to vessel emissions, the ecological impact of shipping documentation, particularly the Bill of Lading (BL), has often been overlooked. Traditional paper-based BLs, critical for international trade, contribute to deforestation, water pollution, and greenhouse gas emissions through their production, transportation, and disposal [3].
As the world grapples with the urgent need to address climate change, the shipping industry's transition to digital solutions presents a significant opportunity for environmental improvement. The emergence of blockchain technology has enabled the development of electronic Bills of Lading (eBL), offering not only enhanced security and efficiency but also the potential for a substantial reduction in paper consumption and associated carbon emissions [4].
This paper aims to quantify the environmental benefits of transitioning from paper BLs to eBLs by comparing their respective carbon footprints. We examine two prominent blockchain technologies for eBLs: public Layer 2 Ethereum using Proof of Stake (PoS) and private blockchain based on Bitcoin using Proof of Work (PoW). Employing a comprehensive Life Cycle Assessment (LCA) approach [5], we analyse the environmental impacts from creation to final use of both paper and electronic BLs.
Our paper addresses the following environmentally focused questions:
What is the total carbon footprint and resource consumption associated with the lifecycle of traditional paper BL?
To what extent can the adoption of eBLs reduce greenhouse gas emissions and resource depletion compared to paper BLs?
How do the environmental impacts of PoS and PoW blockchain technologies compare in the context of eBL systems?
What are the broader ecological implications of widespread eBL adoption in the shipping industry?
By providing a detailed environmental impact assessment, this paper aims to inform sustainable decision-making in the shipping industry. Our findings will offer valuable insights for industry stakeholders, policymakers, and environmental advocates seeking to minimise the ecological footprint of international trade documentation. Moreover, this research contributes to the growing body of knowledge about the environmental potential of digital technologies in traditional industries. As the world seeks innovative solutions to combat climate change [6], understanding the environmental implications of digitising crucial trade documents becomes paramount. This paper not only quantifies the potential ecological benefits of eBLs but also highlights the importance of choosing environmentally conscious technological solutions in the ongoing digital transformation of global trade. In the context of this paper, the term ‘eBL' (electronic Bill of Lading) specifically refers to a blockchain-based digital document, leveraging distributed ledger technology to ensure security, immutability, and seamless transferability in international trade transactions.
2. Understanding the carbon footprint: Its significance and impact
The carbon footprint is an important measure of the environmental impact of individuals, companies, and nations. It represents the total amount of greenhouse gas emissions (mainly carbon dioxide, CO₂) caused by our actions. These emissions contribute significantly to global warming, drive climate change, and lead to consequences such as rising sea levels, extreme weather events, and the destruction of ecosystems [7].
This measure is crucial for several reasons:
1. Environmental impact assessment: It helps us understand the environmental impact of our daily activities. By quantifying emissions from activities such as driving, heating, and production, we can identify the main sources of greenhouse gases (GHG) and take steps to reduce them. For example, the production and consumption of goods and services contribute significantly to our carbon footprint, including emissions from international transport and shipping [8].
2. Resource management: Measuring the carbon footprint is essential for resource management. It enables companies and governments to identify areas where energy consumption can be reduced, leading to cost savings and improved efficiency. For example, switching to renewable energy sources and improving energy efficiency in industrial processes can significantly reduce emissions [9].
3. Political decisions: Carbon footprint data is crucial for policy making. Governments use this information to enact regulations and policies aimed at reducing emissions and promoting sustainable practices. This includes setting emission reduction targets and introducing carbon pricing mechanisms to incentivise lower emissions [10].
4. Awareness and responsibility: Understanding our carbon footprint raises awareness and promotes a sense of responsibility. It helps individuals and organisations make more informed decisions that are better for the planet. For example, by choosing public transport over your own car or supporting businesses with sustainable practices, you can reduce your carbon footprint [11].
The climate crisis has become one of the most pressing problems of the 21st century. According to the International Energy Agency (IEA), global energy-related CO₂ emissions reach a record high of 37.4 billion metric tonnes in 2023, an increase of 1.1% compared to the previous year [12]. This trend is being driven by factors such as the economic upturn and rising demand for energy.
The Paris Agreement, which was adopted in 2015, is the cornerstone of global efforts to combat climate change. It sets out long-term goals to guide all nations to significantly reduce global greenhouse gas emissions to keep the global temperature increase to well below 2°C above pre-industrial levels and to pursue efforts to limit it to 1.5°C above pre-industrial levels, recognising that this would significantly reduce the risks and impacts of climate change [13]. The Intergovernmental Panel on Climate Change (IPCC) published a report on the 1.5ºC target in October 2018 [14]. Projections indicate that without significant mitigation measures, greenhouse gas emissions will continue to rise and could lead to a temperature increase of 3.2°C by 2100. In order to limit global warming to 1.5°C, emissions must be reduced by at least 43% by 2030 compared to 2019 levels [15].
In response to this urgent challenge, many companies are setting themselves ambitious targets, such as achieving carbon neutrality and net zero emissions. This requires reducing emissions wherever possible and offsetting the remaining emissions. Although technologies in various sectors can significantly reduce emissions, achieving zero remains a challenge. A concrete example of such efforts is the switch from paper-based BLs to eBLs, which helps to reduce emissions and achieve these sustainability goals [16].
3. Purpose and scope of the carbon footprint calculation for paper and electronic bills of lading
The purpose of this paper is to provide a comprehensive comparison of the carbon footprint associated with the issuance and transfer of paper and electronic BLs. As the shipping industry increasingly adopts digital solutions, it is crucial to understand the environmental impact of these changes. This comparison aims to highlight the potential carbon savings and environmental benefits of moving from traditional paper-based documents to electronic formats. To substantiate these claims, we have calculated the carbon footprint for both the paper and electronic versions, clearly demonstrating the environmental benefits of eBL.
To determine the environmental impact or carbon footprint of both the paper and electronic BL, we applied the life cycle assessment (LCA) method [5]. This comprehensive approach includes emissions from production, transport, use, and disposal at the end of the life cycle. The LCA analysis helps us to understand the entire environmental footprint of paper and electronic BL and to identify phases in which the impact can be reduced. The paper uses the LCA method to ensure a comprehensive assessment of the environmental impact throughout the life cycle of both types of documents [5].
In addition, this paper explores the wider implications of adopting electronic eBLs, including potential cost savings and efficiency gains in the shipping industry [17], the role of digital transformation in achieving sustainability goals in the maritime sector [18], and the challenges and opportunities presented by the transition to digital documentation [4]. The results presented here are intended to inform decision-makers about the benefits of switching to digitalisation and a more sustainable documentation process in international trade. To achieve the objectives of this paper, we have relied on research from academic journals as well as from available technical and environmental information. All of this information was gathered from public documents. The paper is structured as follows:
An explanation of what a BL is and the role it plays in logistics.
A comparison of paper and electronic versions of BLs.
A presentation of the methodology for calculating the carbon footprint for both types.
Results of the carbon footprint calculations for paper and electronic BLs.
A comparison of the two results and a discussion of the findings.
It is important to note that while we have endeavoured to be accurate and complete, it may be difficult to obtain some data points with absolute precision. In such cases, we have used the most relevant and reliable data available. Similarly, we have focused on the most important aspects of emissions in our calculations, recognising that it may not be possible to account for every minute detail of the process.
4. Paper vs. electronic bill of lading in logistics
The BL is still an important document in international trade and fulfils three basic functions:
Cargo receipt: it serves as proof that the carrier has taken delivery of the goods from the shipper and contains information on the condition and quantity of the cargo at the place of loading. Document of title: The BL serves as a document of title (entitlement to claim cargo incorporated in the bill of lading at the port of destination or to transfer the entitlement if BL is to order). Evidence of the contract of carriage: It embodies or evidences the terms of the contract between the shipper and the carrier for the carriage of goods. This function provides legal protection for both parties, usually under international conventions such as the Hague or Hague-Visby. [22].
4.1 Paper bill of lading: Drawbacks
Despite its long history, the paper BL poses some significant challenges in modern international trade. The time-consuming processing, signing, and transport of paper documents across borders and long distances often results in delays in shipping, payments, and transfer of title, which in turn leads to extended shipping times, demurrage, and general inefficiencies in global trade [21, 24]. In addition, paper BLs are inherently vulnerable to security risks such as theft, loss, forgery, and physical damage, with dispute resolution and fraud management processes proving to be time-consuming and costly [25]. From an environmental perspective, the use of paper contributes to deforestation and pollution, which is exacerbated by the additional emissions generated by the global transport of these documents [3, 20]. In addition, paper-based systems are unable to track shipments in real time, making it difficult to provide up-to-date information on the status of shipments and documents [26]. Finally, manual data entry and paper-based record-keeping associated with paper BL systems are error-prone and inefficient, impacting the overall efficiency of trade operations [24]. These multifaceted challenges emphasise the need for more efficient, secure, and environmentally friendly alternatives in the field of international trade documentation.
4.2 Blockchain-based electronic bill of lading (eBL): A modern solution
Blockchain-based eBLs offer significant advances over traditional paper-based systems in global trade [24]. These digital solutions increase efficiency by enabling the instantaneous transfer of documents, drastically reducing delays in shipping processes [4, 26]. This acceleration also extends to title transfers, authorisations, and payments, significantly reducing shipping delays and increasing the overall speed of trade [24]. Real-time tracking and transparency are the key features of eBLs. They allow all parties to access up-to-date information at the same time, avoiding disputes and improving logistics efficiency [24, 27, 28]. The digital nature of eBLs significantly reduces the administrative burden by eliminating manual processing and integrating smart contracts that automate crucial steps in the shipping process [29]. In addition, eBLs contribute to environmental sustainability by reducing paper consumption and the associated transport, thus reducing the carbon footprint of global trade documentation [25]. Although the initial implementation requires investment, the long-term cost savings can be significant due to reduced paper usage, faster turnaround times, and lower error rates, making a compelling business case for adoption [27]. Blockchain-based eBLs solve many of the problems of paper eBLs and offer a faster, more secure, and environmentally friendly alternative that promises to revolutionise international trade [30, 31].
5. Methodology for calculating the carbon footprint
The carbon footprint of a product or process measures the total greenhouse gases emitted both directly and indirectly during its life cycle. For BL, whether paper or electronic, this includes the assessment of emissions from the production, use, and disposal phases.
For paper BL, we have used data from UK government conversion factors [10] to assess emissions from paper production, printing, transport, and disposal. For the electronic version, we analysed data from our platform from 2023, focusing on the emissions generated by processing transactions and energy consumption from cloud computing services, particularly Amazon Web Services (AWS). The scope of this analysis includes examining the carbon emissions generated throughout the lifecycle of both paper and electronic waybills. This includes:
Production phase: For paper BLs, this includes the emissions associated with paper production, printing, and the production of envelopes. For eBLs, this includes the energy consumption of the digital infrastructure required to create and manage the electronic documents [17].
Distribution phase: This includes the emissions generated by the physical transport of paper BLs and the data transmission requirements for eBLs [18].
Utilisation phase: This phase includes the emissions associated with handling, storing, and accessing BLs in paper and electronic form [19].
End-of-life phase: For BLs in paper form, this includes the emissions associated with disposal or recycling. For eBLs, this includes the energy required for the secure deletion or archiving of digital data [19].
The unit used in our calculation is the carbon dioxide equivalent (CO₂e), which represents the combined effect of all greenhouse gases on global warming. While carbon dioxide is the most abundant and impactful gas, it is not the only one that contributes to climate change. CO₂e simplifies the measurement by converting the impact of the various greenhouse gases into a carbon dioxide equivalent. This approach ensures that the contribution of each gas is accurately represented, as they all play a significant role in climate change, even though carbon dioxide remains the largest contributor [32].
It is important to emphasise that it is difficult to obtain some data with absolute precision. In such cases, we have collected the most relevant data available and made reasonable assumptions based on industry standards and peer-reviewed literature. Similarly, we have not considered all aspects of emissions in the calculation and have instead focused on the most important factors contributing to the carbon footprint. However, due to insufficient data availability and limited meaningful results, we have decided not to include the utilisation phase for paper BL in our LCA analysis for the purposes of this paper.
In addition, the indirect emissions associated with the manufacture and life cycle of the information technology infrastructure (computers, monitors, printers) were excluded from the comparative analysis, as these capital goods represent a common denominator in the paper-based and electronic BL processing processes, and thus their relative impact is neutralised in the differentiated assessment.
To ensure a fair comparison, we have calculated the carbon footprint for a single BL in both paper and electronic form. This allows a direct comparison of the environmental impact of each method on the basis of a single document. For the purposes of this study, a standard paper BL was defined as a single A4 sheet printed on both sides.
To account for the variability of certain parameters (e.g., transport distances for paper BLs or energy mix for data centres hosting eBLs), we performed sensitivity analyses where appropriate. This helps to identify a range of possible outcomes and determine which factors have the greatest impact on the overall carbon footprint.
The main objective of this study is to analyse, calculate, and evaluate the environmental impact of eBLs compared to their paper counterpart. The conclusion summarises the results and shows how the eBL service helps to reduce the carbon footprint and promote sustainability in the shipping industry.
6. Carbon footprint of a paper BL
The production of BL paper involves several steps, from the extraction of raw materials to their disposal or recycling. Each step contributes to the overall carbon footprint of the document. A comprehensive life cycle assessment of paper BL was carried out for this paper [5]. The assessment covered material sourcing and production of the document, including paper manufacturing, which requires significant use of water, energy, and chemical resources; document production, including emissions associated with computer use; and the printing process, which took into account paper consumption, ink consumption, and printer-related emissions. The LCA also considers the distribution of the documents and analyses the emissions generated by the transport of the physical document. In addition, the assessment covered the use and life cycle of the document, i.e., the functional period limited to the verification of the original document, the post-verification phase where the recipient collects the specified goods, the dormant phase of archiving, and the final phase culminating in the final destruction of the document. This LCA framework provides a comprehensive basis for quantifying the environmental impacts associated with paper BLs throughout their life cycle, from production to disposal.
In the calculation procedure for the creation of a BL paper, a paper weight of 80 g/m² and an A4 sheet format (210 x 297 mm, 0.062 m²) were assumed for the weight calculation, which results in a weight of an A4 sheet of 4.96 g. The CO₂ emissions for BL production were calculated with an emission factor of 0.911 kg CO₂e per kilogram of paper [10], which results in 4.52 g CO₂e per sheet. The preparation, data entry, and printing processes were also taken into account, with data entry and document preparation using a computer resulting in 17.57 g CO₂e emissions [39]. The estimated emissions for printing were 1 g/page for a laser printer [23] or 2 g for double-sided printing. The total emission for the production of paper BL was calculated at 24.09 g CO₂e.
For the calculation of CO₂e emissions during BL transport, air transport was taken into account as it is often used for time-sensitive documents in international trade, although it is the most emission-intensive method. The input data included an envelope weight of 90 g [35] and the weight of a paper BL of 4.96 g, totalling 0.09496 kg. The emission factor associated with the production of cardboard envelopes was quantified as 0.80152 kg CO₂e emissions per kilogram of product weight. The emissions of the envelope and a sheet of paper were calculated at 76.66 g CO₂e [10]. It should be noted that the envelope usually contains additional original documents that are important for international trade transactions, which can increase the total weight and emissions.
Transport distance calculation: To ensure optimal credibility of our calculations, we will apply a systematic approach to measuring distances between selected global maritime transport hubs. Our methodology involves the selection of 15 strategically chosen airports in close proximity to major seaports spread across different geographical regions worldwide. For each pair of these locations, we calculate the great circle distance, which is the shortest path between two points on the surface of a sphere, using the Haversine formula. It is important to note that this method is an approximation of the distances of air travel if the aircraft were to fly from point to point. While the Haversine formula does not take into account the ellipsoidal shape of the Earth, it provides a computationally efficient and sufficiently accurate method for our purposes, with errors typically less than 0.5% over most distances on the Earth's surface.
Significant ports by geo region:
- North America: Los Angeles, New York
- South America: Santos (Brazil), Buenos Aires (Argentina)
- Europe: Rotterdam (Netherlands), Hamburg (Germany)
- Africa: Durban (South Africa), Lagos (Nigeria)
- Asia: Shanghai (China), Singapore
- Middle East: Jebel Ali (UAE)
- Australia: Sydney (Australia)
- Indian Subcontinent: Mumbai (India)
- East Asia: Busan (South Korea), Yokohama (Japan)
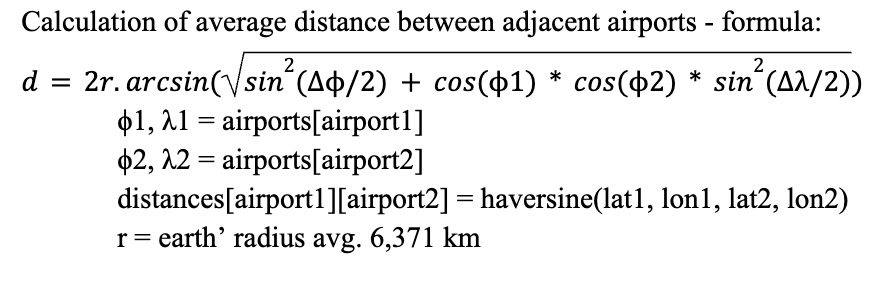
Matrix of air distances between major global ports' airports
Note: The matrix calculates direct air distances and does not take into account actual flight paths, which may be longer due to various factors such as air traffic control restrictions, weather conditions, and any other factors.
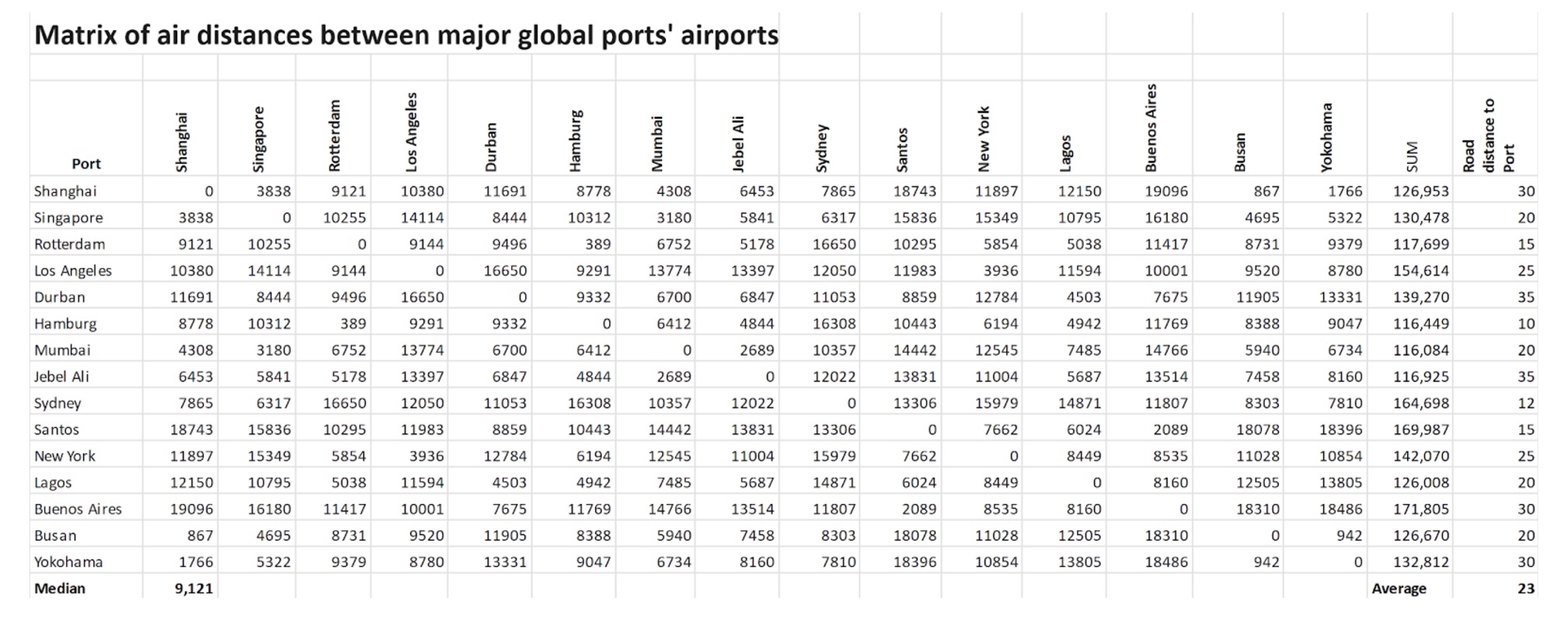
The median airline distance between airports adjacent to selected ports worldwide is determined from a matrix calculated with the above model and is 9,121 km.
In addition, we calculated the average distance for road transport from the nearest airport to the seaport, which was found to be 23 kilometres.
Two different methods were used to assess the carbon emissions associated with paper BL in order to ensure objective quantification. The first, the so-called GHG factor method, calculates the emissions as the product of the weight of the BL envelope, the distance travelled, and a specific emission factor. This approach led to a result of 951.90 g CO₂e [36]. The second method, which was based on fuel consumption emissions calculations, used an Airbus A321 P2F model and took into account factors such as maximum payload, flight duration, and fuel capacity rates. This method resulted in an emissions estimate of 801.87 g CO₂e [37]. In order to reconcile these differing results, the median value of 876.89 g CO₂e was adopted as the final calculation.
When further refining the emissions estimate, the emissions from land transportation from the airport to the seaport were added, which covers a distance of 23 km and causes an additional 0.398 g CO₂e. Consequently, the total transport emissions were calculated at 877.29 g CO₂e.
The emissions from the disposal and end-of-cycle phases were quantified using an estimated factor that takes into account the pulping process during paper recycling. In this calculation, the recycling emission factor (0.02128 kg CO₂e / kg) was multiplied by the weight of the shipment (0.09496 kg), resulting in 2.02 g CO₂e [10] emissions associated with the end-of-life phase of the document.
To determine the comprehensive carbon footprint of a paper BL, the emissions from all phases of its life cycle were added together. This included emissions from paper production (76.66 g CO₂e), document preparation (19.57 g CO₂e), transportation (877.29 g CO₂e) and disposal (2.02 g CO₂e). The total CO₂e footprint, including all phases per paper BL, was calculated at 975.54 g CO₂e.
This LCA shows that there is a significant environmental impact throughout the life cycle of a paper BL. Each phase contributes to greenhouse gas emissions, energy and/or water consumption, air and water pollution, and waste generated by the production and printing processes.
Putting these findings in the context of the global trade industry, the scale of the problem becomes clear. It is estimated that 28.5 billion paper trade documents are used annually [38], with shipping companies alone issuing around 45 million bills of lading per year [34]. These figures underline the significant contribution to paper consumption and associated emissions in the global trade sector and highlight the potential environmental benefits that could be achieved through the adoption of digital alternatives.
The comprehensive nature of this analysis, which incorporates multiple calculation methods and considers different lifecycle stages, provides a solid foundation for understanding the environmental impact of paper-based documents in global trade. It also provides valuable insights for policy makers and industry representatives considering a transition to more sustainable practices in international trade documentation.
7. Carbon footprint of electronic bill of lading
The digitalisation of maritime documentation through electronic bills of lading (eBL) represents a significant technological development in the shipping industry. This chapter presents a quantitative analysis of the environmental impact of eBL systems, specifically examining the different environmental impacts of two predominant blockchain architectures: public Ethereum on layer 2, which implements a Proof of Stake (PoS) consensus, and private blockchain networks using Bitcoin-derived Proof of Work (PoW) protocols.
A basic understanding of the underlying environmental metrics of Layer 1 networks is a prerequisite for evaluating their respective Layer 2 implementations. The Bitcoin network, which operates with a PoW consensus, has an annual energy consumption of about 130 TWh [40], resulting in a carbon footprint of 350-400 kg CO₂e per transaction [41]. In contrast, the Ethereum Layer 1 network has a significantly lower energy requirement after the switch to the PoS architecture, which manifests itself in a carbon footprint of 0.1-0.2 kg CO₂e per transaction [42].
Layer 2 solutions deployed on Ethereum's PoS infrastructure exhibit improved scalability coefficients and lower transaction costs compared to the primary chain while maintaining minimal energy consumption profiles through PoS consensus mechanisms [28]. These implementations enable public verification protocols and transaction transparency while maintaining comprehensive interoperability within the Ethereum ecosystem. Empirical analysis of layer 2 public Ethereum implementations, particularly the Polygon network, shows exceptionally low emission metrics with a carbon footprint per transaction of 0.0003 g to 0.4 g CO₂e [43].
Private blockchain networks implementing Bitcoin-derived PoW consensus mechanisms offer increased security through computationally intensive processes while enabling comprehensive network control for the operator. While these systems facilitate customisation for specific industrial applications, their architecture limits public auditing capabilities due to restricted network access. These private PoW implementations have varying but consistently elevated energy consumption profiles and generate estimated carbon footprints of 10–50 kg CO₂e per transaction, which are lower than those of the public Bitcoin network due to the smaller network size but still represent a significant environmental impact [41].
This research uses the LCA method to quantify the carbon footprint of electronic eBL solutions [5]. The analytical framework includes blockchain transaction processes, cloud computing operations and the usage behaviour of end-user devices. The methodology incorporates data derived from Ethereum Layer 2 public statistical analyses, Ethereum Foundation reports [42], private Bitcoin-based blockchain estimates [40, 41], cloud computing emission metrics from major infrastructure providers, and published LCA data for end-user devices [39].
The cloud computing operations associated with document preparation and storage generate approximately 17.15 mg CO₂e, which remains the same for all blockchain implementations [44]. Emissions from device usage were quantified based on standardised 30-minute operating times for eBL processing, using an Apple MacBook Pro 14" as a reference device. The analysis of the device's 4-year life cycle assessment of 225 kg CO₂e [39] results in calculated hourly operating emissions of 35.15 g CO₂e.
A comprehensive environmental impact assessment of the eBL implementation required the evaluation of the entire operational lifecycle, which includes document creation, blockchain transactions, cloud computing processes, and document delivery protocols. Quantitative analysis of the carbon footprint for an eBL using the Polygon Layer 2 solution reveals the following components:
- Document creation (30-minute device usage): 17.575 g CO₂e
- Polygon blockchain transaction processing: 0.00324 g CO₂e
- Cloud computing operations: 0.01715 g CO₂e
- Operational life cycle (use, storage, deletion): 17.575 g CO₂e
Total carbon footprint: 35.17 g CO₂e
A comparative analysis shows that private Bitcoin-based eBL implementations cause significantly higher emissions due to the PoW consensus requirements, with the blockchain transaction components alone causing an additional 10-50 kg of CO₂e per document. This significant difference in emissions metrics shows that public layer 2 solutions utilising Ethereum's PoS network are significantly more environmentally friendly.
8. Results overview
Our analysis shows that the use of eBL leads to a significant reduction in CO₂e emissions compared to traditional paper methods [43]. Quantitatively, a traditional paper eBL generates about 975.54 g CO₂e, while an eBL using a layer 2 public Ethereum solution (PoS) generates only about 35.17 g CO₂e. This represents a remarkable reduction in carbon emissions of almost 96% [28, 44].
However, it is important to note that the environmental benefits of eBL systems are not the same for all implementations [41]. While the public Ethereum solution on layer 2 (PoS) generates about 35.17 g CO₂e per eBL, private Bitcoin-based (PoW) implementations can generate emissions between 10.05 and 50.05 kg CO₂e per eBL [41, 42]. This stark contrast underlines the crucial importance of technological choices in determining environmental impact.
Switching from paper BL to eBL using PoS blockchain can reduce carbon emissions by up to 96%, highlighting the significant potential for emission reduction in the shipping industry [43]. The choice of blockchain technology has a significant impact on the environmental benefits of eBLs, with PoS systems having significantly lower emissions compared to PoW systems [28].
It is important to note that in some scenarios, poorly implemented or energy-intensive eBL systems could potentially emit more CO₂e than traditional paper systems, highlighting the need for careful system design and implementation [41]. While both eBL systems offer advantages over paper-based methods in terms of efficiency and safety, these advantages must be weighed against their environmental impact [45].
Scalability considerations also play a role in the overall environmental impact. Layer 2 solutions on Ethereum generally offer better scalability compared to private PoW networks, which can face challenges as the network grows [42]. In addition, public layer 2 solutions on Ethereum offer better integration into the broader blockchain ecosystem, which could be beneficial for organisations looking to leverage blockchain technology in different business areas [46, 47].
The public Ethereum solution on Layer 2, which utilises the energy-efficient PoS consensus mechanism, provides a balance of energy efficiency, scalability, and transparency [47]. This finding has significant implications for the shipping industry and policy makers. It suggests that the adoption of PoS-based eBL systems could play a crucial role in reducing the carbon footprint of global trade documentation processes.
In summary, while eBL systems generally offer significant environmental benefits over traditional paper-based methods, the specific implementation and underlying blockchain technology significantly influence the extent of these benefits. Future research and development in this area should focus on optimising eBL systems in terms of both operational efficiency and environmental sustainability.
9. Conclusion
The transition from paper-based to electronic bills of lading represents a significant step forward for the shipping industry, offering improvements in efficiency, safety, and environmental sustainability. Our research provides compelling evidence that the adoption of eBL systems can lead to a significant reduction in carbon emissions, particularly when energy-efficient blockchain technologies are used.
The environmental impact of eBL systems varies greatly depending on the underlying blockchain technology. Systems that use public layer 2 Ethereum with Proof of Stake (PoS) can reduce carbon emissions by over 90% compared to traditional paper eBLs, making a significant contribution to sustainability efforts. However, eBL systems based on private Bitcoin-based Proof of Work (PoW) blockchains present a more complex scenario. While they offer the digital advantages of eBLs, their high energy consumption can negate the environmental benefits and, in some cases, even exceed the emissions of paper-based systems.
These results show how important it is to carefully consider the technological options when implementing eBL systems. Public Layer 2 Ethereum solutions offer superior energy efficiency and scalability, making them an attractive option for organisations that value environmental sustainability. Conversely, private Bitcoin-based solutions offer more privacy and control, which may be crucial for certain organisations or in certain regulatory environments. This trade-off between environmental impact and other operational priorities is an important consideration that industry players need to weigh carefully.
The implications of these findings for the shipping industry are significant. As the industry digitises, there is an opportunity to significantly reduce its environmental footprint. However, in order to reap these benefits, informed decisions need to be made about the underlying technologies. Companies should prioritise energy-efficient solutions wherever possible while taking into account their specific needs in terms of data protection, control, and regulatory compliance.
Looking to the future, there is potential for further innovation in this area. The development of hybrid approaches that combine the energy efficiency of PoS systems with the privacy features of private blockchains could be a best-of-both-worlds solution. In addition, companies that opt for private PoW solutions due to specific operational requirements could offset the higher energy consumption by investing in renewable energy sources.
The important part of the shipping industry's commitment to switch completely to electronic bills of lading by 2030 is a promising step forward. This transition promises not only environmental benefits but also improvements in cost reduction and operational efficiency. As blockchain technologies continue to evolve, we can expect to see further advances in the energy efficiency, scalability, and functionality of eBL systems.
In summary, while there are clear environmental benefits to adopting eBL systems, particularly when energy- efficient technologies are used, the industry needs to carefully consider the trade-offs between the various blockchain solutions. By making informed decisions, continuing to innovate, and prioritising sustainability alongside other operational needs, the shipping industry can make an important contribution to global efforts to reduce carbon emissions while improving the efficiency and security of international trade. The way forward requires continuous assessment, openness to new technologies, and a commitment to balancing environmental responsibility with operational requirements. As the world grapples with the urgent need to combat climate change, the shipping industry's transition to more sustainable practices, including the adoption of environmentally friendly eBL systems, will play a crucial role in building a more sustainable future for global trade.
10. References:
[1] United Nations Conference on Trade and Development (UNCTAD). (2023). Review of Maritime Transport 2023. Retrieved from https://unctad.org/system/files/official-document/rmt2023_en.pdf (Accessed September 16, 2024).
[2] International Maritime Organization (IMO). (2023). Fourth IMO GHG Study 2020. Retrieved from https://www.imo.org/en/OurWork/Environment/Pages/Fourth-IMO-Greenhouse-Gas-Study-2020.aspx (Accessed September 16, 2024).
[3] Nazaruk, Z. (2020). ‘Is digital more environmentally friendly than paper? - Except Integrated Sustainability B.V.’ (2020) Retrieved from https://except.eco/knowledge/is-digital-more-environmentally-friendly-than-paper/ (Accessed September 16, 2024).
[4] Yang, C. S. (2019). Maritime shipping digitalization: Blockchain-based technology applications, future improvements, and intention to use. Transportation Research Part E: Logistics and Transportation Review, 131, 108-117. https://www.researchgate.net/publication/336962836_Maritime_shipping_digitalization_Blockchain-based_technology_applications_future_improvements_and_intention_to_use (Accessed September 19, 2024).
[5] ISO 14040:2006. (2006). Environmental management — Life cycle assessment — Principles and framework. International Organization for Standardization. https://www.iso.org/standard/37456.html (Accessed September 16, 2024).
[6] Intergovernmental Panel on Climate Change (IPCC). (2023). Climate Change 2023: Synthesis Report. Retrieved from https://www.ipcc.ch/report/ar6/syr/ (Accessed September 16, 2024).
[7] Selin, N. E. (2024). Carbon footprint. In Britannica. Retrieved from https://www.britannica.com/science/carbon-footprint (Accessed September 16, 2024).
[8] Wiedmann, T., & Minx, J. (2008). A Definition of 'Carbon Footprint'. In: C. C. Pertsova, Ecological Economics Research Trends: Chapter 1, pp. 1-11, Nova Science Publishers, Hauppauge NY, USA. https://www.researchgate.net/publication/247152314_A_Definition_of_Carbon_Footprint (Accessed September 16, 2024).
[9] Gibson, K. (2024). How businesses can measure & reduce carbon emissions. Harvard Business School Online. Retrieved from https://online.hbs.edu/blog/post/how-to-reduce-carbon-emissions (Accessed September 16, 2024).
[10] UK Government. (2021). Valuation of greenhouse gas emissions: For policy appraisal and evaluation. Retrieved from https://www.gov.uk/government/publications/valuing-greenhouse-gas-emissions-in-policy-appraisal/valuation-of-greenhouse-gas-emissions-for-policy-appraisal-and-evaluation (Accessed September 16, 2024).
[11] Enel Green Power. (2023). What is the carbon footprint and how is it calculated? Retrieved from https://www.enelgreenpower.com/learning-hub/energy-transition/carbon-footprint (Accessed September 17, 2024).
[12] International Energy Agency. (2024). CO2 emissions in 2023. IEA Publications. Retrieved from https://www.iea.org/reports/co2-emissions-in-2023/executive-summary (Accessed September 17, 2024).
[13] United Nations. (n.d.). The Paris Agreement. Retrieved from https://www.un.org/en/climatechange/paris-agreement (Accessed September 17, 2024).
[14] Intergovernmental Panel on Climate Change (IPCC). (2018). Global warming of 1.5°C: An IPCC special report on the impacts of global warming of 1.5°C above pre-industrial levels and related global greenhouse gas emission pathways. Retrieved from https://www.ipcc.ch/sr15/ (Accessed September 17, 2024)
[15] Cooper, N. L. (2023). The IPCC just published its summary of 5 years of reports - Here's what you need to know. UNFCCC. Retrieved from https://climatechampions.unfccc.int/the-ipcc-just-published-its-summary-of-5-years-of-reports-heres-what-you-need-to-know/ (Accessed September 16, 2024).
[16] Digital Container Shipping Association (DCSA). (2023). DCSA's member carriers commit to a fully standardized electronic Bill of Lading by 2030. Retrieved from https://dcsa.org/newsroom/dcsas-member-carriers-commit-to-a-fully-standardised-electronic-bill-of-lading-by-2030/ (Accessed September 16, 2024).
[17] Tenhunen, M., & Penttinen, E. (2010). Assessing the carbon footprint of paper vs. electronic invoicing. ACIS 2010 Proceedings, 95. Retrieved from https://aisel.aisnet.org/acis2010/95/ (Accessed September 19, 2024).
[18] McKinnon, A. (2010). Product-level carbon auditing of supply chains: Environmental imperative or wasteful distraction? International Journal of Physical Distribution & Logistics Management, 40(1/2), 42-60. Retrieved from https://www.emerald.com/insight/content/doi/10.1108/09600031011018037/full/html (Accessed September 19, 2024).
[19] Pihkola, H., Nors, M., Kujanpää, M., Helin, T., Kariniemi, M., Pajula, T., Dahlbo, H., & Koskela, S. (2010). Carbon footprint and environmental impacts of print products from cradle to grave. VTT Technical Research Centre of Finland. Retrieved from https://publications.vtt.fi/pdf/tiedotteet/2010/T2560.pdf (Accessed September 19).
[20] Czachorowski, K., Solesvik, M., & Kondratenko, Y. (2019). The Application of Blockchain Technology in the Maritime Industry. In Green IT Engineering: Social, Business and Industrial Applications (pp. 561-577). Springer, Cham. Retrieved from https://www.researchgate.net/publication/327975787_The_Application_of_Blockchain_Technology_in_the_Maritime_Industry (Accessed September 19, 2024).
[21] Goldby, M. (2013). Electronic bills of lading and central registries: what is holding back progress? Information & Communications Technology Law, 22(2), 125-149. Retrieved from https://www.researchgate.net/publication/240524954_Electronic_bills_of_lading_and_central_registries_what_is_holding_back_progress (Accessed September 16, 2024).
[22] Green, I., & Vasiliou, R. (2018). The Hague Visby Rules, Contracts of carriage and bills of lading. The Standard Club Ltd. Retrieved from https://www.standard-club.com/fileadmin/uploads/standardclub/Documents/Import/publications/goto-handouts/2767683-contracts-of-carriage-and-bills-of-lading-the-hague-visby-rules.pdf (Accessed September 16, 2024).
[23] Greenhouse Gas Protocol. (2023). Calculation Tools. Retrieved from https://ghgprotocol.org/calculation-tools (Accessed September 16, 2024).
[24] Chang, Y., Iakovou, E., & Shi, W. (2020). Blockchain in global supply chains and cross border trade: a critical synthesis of the state-of-the-art, challenges and opportunities. Retrieved from
https://doi.org/10.1080/00207543.2019.1651946 (Accessed September 19, 2024).
[25] Tijan, E., et al. (2021). Digital transformation in the maritime transport sector. Retrieved from
https://doi.org/10.1016/j.techfore.2021.120879 (Accessed September 16, 2024).
[26] Jović, M., et al. (2019). An Overview of Security Challenges of Seaport IoT Systems. Retrieved from
https://www.scribd.com/document/411524791/An-Overview-Of-Security-Challenges-Of-Seaport-IoT-Systems-MIPRO-2019-Saša-Aksentijević (Accessed September 16, 2024).
[27] Osmani, M., El-Haddadeh, R., Hindi, N., Janssen, M., & Weerakkody, V. (2021). Blockchain for next generation services in banking and finance: cost, benefit, risk and opportunity analysis. Journal of Enterprise Information Management, 34(3), 884-899. Retrieved from
https://www.emerald.com/insight/content/doi/10.1108/JEIM-02-2020-0044/full/html (Accessed September 17, 2024).
[28] Platt, M., et al. (2021). Energy footprint of blockchain consensus mechanisms beyond proof-of-work. Retrieved from https://arxiv.org/abs/2109.03667. (Accessed September 16, 2024).
[29] Korpela, K., Hallikas, J., & Dahlberg, T. (2017). Digital supply chain transformation toward blockchain integration. Retrieved from https://scholarspace.manoa.hawaii.edu/items/e78aa782-e88a-454d-af76-473f9d276da5 (Accessed September 16, 2024).
[30] Saberi, S., et al. (2019). Blockchain technology and its relationships to sustainable supply chain management. Retrieved from https://www.tandfonline.com/doi/abs/10.1080/00207543.2018.1533261 (Accessed September 17, 2024).
[31] Saleh, F. (2021). Blockchain without waste: Proof-of-stake. The Review of Financial Studies, 34(3), 1156-1190. Retrieved from https://academic.oup.com/rfs/article-abstract/34/3/1156/5868423?login=false (Accessed September 16, 2024).
[32] Climatiq. (2023). Emission factor database. Retrieved from
https://www.climatiq.io/data/emission-factor/289b489d-5425-4574-a77f-096c8713e3b8 (Accessed September 24, 2024)
[33] de Vries, A., & Stoll, C. (2021). Bitcoin's growing e-waste problem.
https://www.sciencedirect.com/science/article/abs/pii/S0921344921005103 (Accessed September 24, 2024)
[34] DCSA. (2023). DCSA’s member carriers commit to a fully standardized electronic Bill of Lading by 2030. Digital Container Shipping Association. Retrieved from https://dcsa.org/newsroom/dcsas-member-carriers-commit-to-a-fully-standardised-electronic-bill-of-lading-by-2030 (Accessed September 16, 2024).
[35] Karton.eu. (n.d.). 308x235x0-30 mm Solid Board Envelope A4 White. Retrieved from
https://www.karton.eu/308x235x0-30-mm-Solid-Board-Envelope-A4-White (Accessed September 16, 2024).
[36] U. Retrieved from
https://www.gov.uk/government/publications/greenhouse-gas-reporting-conversion-factors-2023 (Accessed September 16, 2024).
[37] Airbus. (2023). A320 Freighter Family. Retrieved from
https://aircraft.airbus.com/en/aircraft/freighters/a320-freighter-family (Accessed September 25, 2024).
[38] UK Government. (2022). Impact assessment of the Electronic Trade Documents Bill. Retrieved from https://www.gov.uk/government/publications/electronic-trade-documents-bill-impact-assessment/impact-assessment-of-the-electronic-trade-documents-bill#fn:17 (Accessed September 16, 2024).
[39] Apple Inc. (2023). Product Environmental Report: 14-inch MacBook Pro. Retrieved from
https://www.apple.com/environment/pdf/products/notebooks/14-inch_MacBook_Pro_PER_Oct2023.pdf (Accessed September 16, 2024).
[40] Cambridge Bitcoin Electricity Consumption Index. (2023). Cambridge Centre for Alternative Finance. Retrieved from https://ccaf.io/cbnsi/cbeci (Accessed September 16, 2024).
[41] de Vries, A., & Stoll, C. (2021). Bitcoin's growing e-waste problem. Retrieved from
https://www.sciencedirect.com/science/article/abs/pii/S0921344921005103 (Accessed September 17, 2024).
[42] Ethereum Foundation. (2023). Ethereum Energy Consumption. Retrieved from
https://ethereum.org/en/energy-consumption/ (Accessed September 16, 2024).
[43] Polygon. (2022). Polygon's Green Manifesto: A Smart Contract with Planet Earth. Retrieved from
https://polygon.technology/blog/our-green-manifesto (Accessed September 16, 2024).
[44] Masanet, E., et al. (2020). Recalibrating global data center energy-use estimates. Science, 367(6481), 984-986. Retrieved from https://doi.org/10.1126/science.aba3758 (Accessed September 16, 2024).
[45] Kouhizadeh, M., & Sarkis, J. (2018). Blockchain practices, potentials, and perspectives in greening supply chains. Sustainability, 10(10), 3652. Retrieved from https://doi.org/10.3390/su10103652 (Accessed September 16, 2024).
[46] Saberi, S., et al. (2019). Blockchain technology and its relationships to sustainable supply chain management. International Journal of Production Research, 57(7), 2117-2135. Retrieved from
https://www.tandfonline.com/doi/abs/10.1080/00207543.2018.1533261 (Accessed September 16, 2024).
[47] Saleh, F. (2021). Blockchain without waste: Proof-of-stake. The Review of Financial Studies, 34(3), 1156-1190. Retrieved from https://academic.oup.com/rfs/article-abstract/34/3/1156/5868423 (Accessed September 17, 2024).